2022-08-28 12:55-Science Material Station
First author:Lian-Sheng Li
Correspondence should be addressed to YUAN-FU DENG*.
Unit:South China University of Technology
【Research Background】
All-solid-state lithium metal batteries are expected to achieve higher safety and energy density than current commercial liquid lithium-ion batteries. However, notorious interfacial issues, such as insufficient interfacial contacts, interfacial side reactions, and uneven lithium deposition on the anode side, severely limit the performance of the assembled all-solid-state batteries. Among many interfacial modification strategies, because a small amount of functional additives can achieve significant modification effects, it is a simple and low-cost method to introduce functional additives into composite solid electrolytes. It has received increasing attention from researchers.
【Introduction】
Recently, Professor Deng Yuanfu from South China University of Technology published a paper titled “Optimized Functional Additive Enabled Stable Cathode and Anode Interfaces for High-voltage All-Solid-State Lithium Batteries with Significantly Improved Cycling Performance” in the internationally renowned journal Journal of Materials Chemistry A research papers.
This paper systematically revealed the effect of lithium difluorooxalate borate (LiDFOB) and lithium difluorodioxalate phosphate (LiBODFP) functional additives on the positive and negative interfaces in all-solid-state lithium metal batteries, and proposed that it can The strategy of adding different functional additives to achieve ultra-stable cycling of all-solid-state lithium metal batteries.
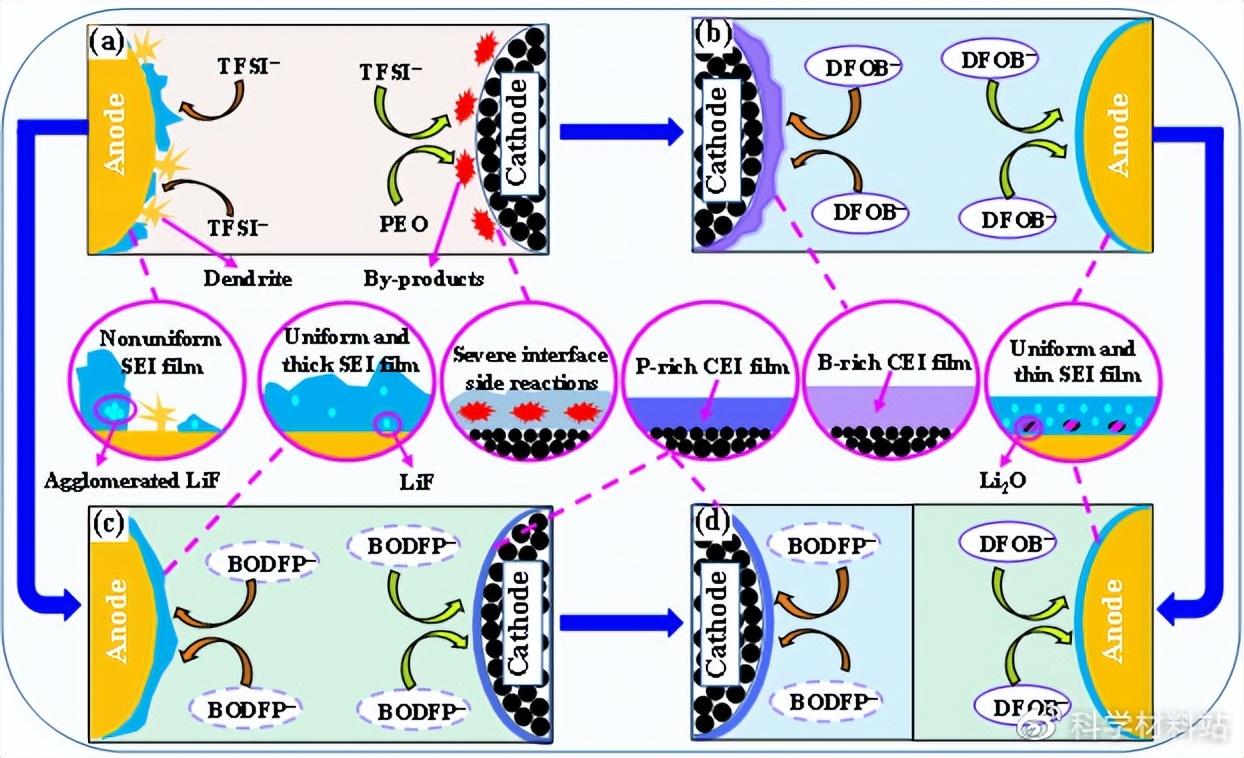
Fig. 1. Schematic diagram of the effect of different functional additives on the positive and negative interface in all-solid-state lithium metal batteries.
【Key points of this article】
Key point 1: The influence of different additives on the cycle performance of all-solid-state batteries
The long-term cycle performance of all-solid-state batteries assembled with electrolyte membranes containing different functional additives and polycrystalline NCM622 at 1.0 C showed significant differences. Specifically, the capacity retention rate of the battery is LiBODFP>LiDFOB>additive-free. By analyzing the corresponding charge and discharge curves and the AC impedance results after different cycles, it can be concluded that the battery capacity fading is mainly caused by the instability of the positive electrode/electrolyte interface.
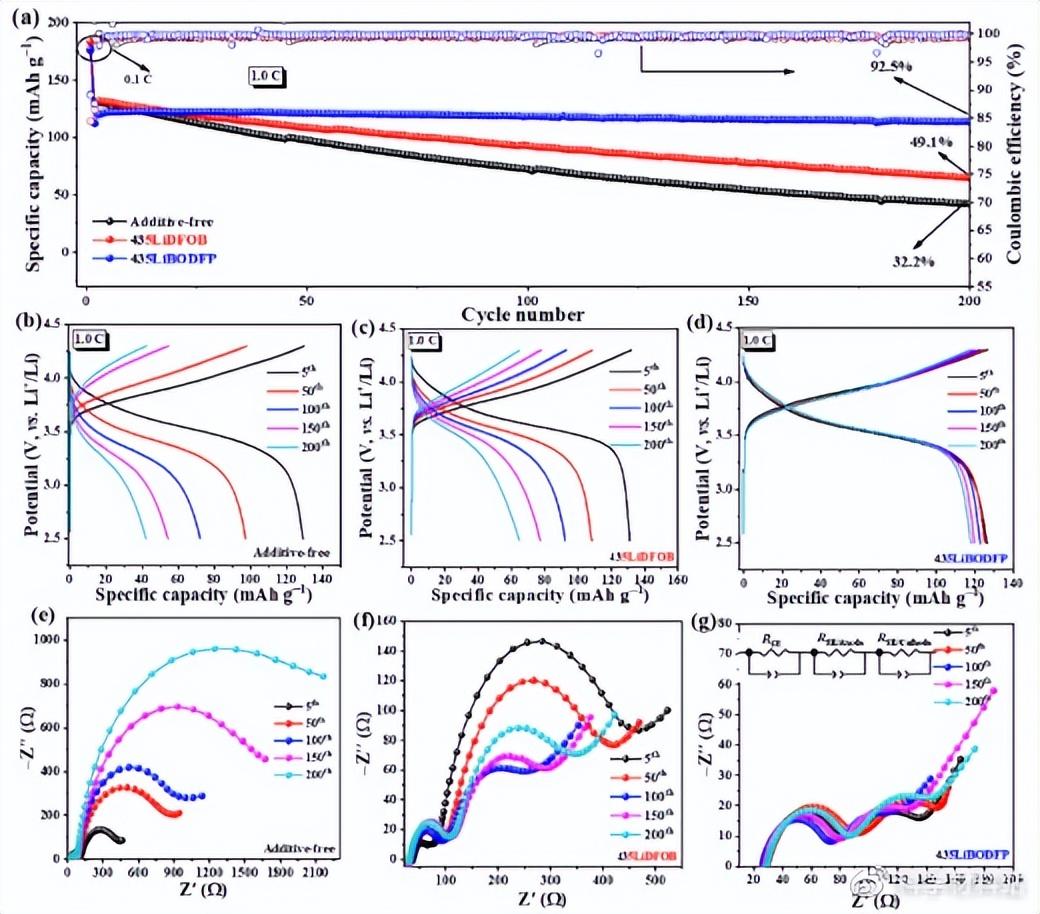
Fig. 2. (a) Cycling stability of batteries assembled with composite electrolyte membranes with different additives in the voltage range of 1.0 C and 2.5-4.3 V; charge-discharge curves of the assembled batteries after different cycles: (b) without additives , (c) 435LiDFOB and (d) 435LiBODFP composite electrolytes, and EIS results of batteries assembled from (e) no additives, (f) 435LiDFOB and (g) 435LiBODFP composite electrolytes after different cycles
Point 2: Functional additives can inhibit side reactions of positive and negative electrodes
The cycled battery was disassembled and the electrodes were characterized. XRD results proved that severe interfacial side reactions occurred on the positive side of the battery without additives. Surprisingly, obvious side reaction products (especially Li4B2O5) were also observed on the cathode side of the LiDFOB-containing battery, which indicates that the oxidative decomposition of LiDFOB is more significant. In contrast, side reactions at the cathode interface of batteries containing LiBODFP are significantly suppressed. Furthermore, the morphology of the Li metal surface after cycling and the air exposure experiments prove that LiDFOB and LiBODFP can be reduced to form a film on the Li surface, thereby protecting the Li metal anode.
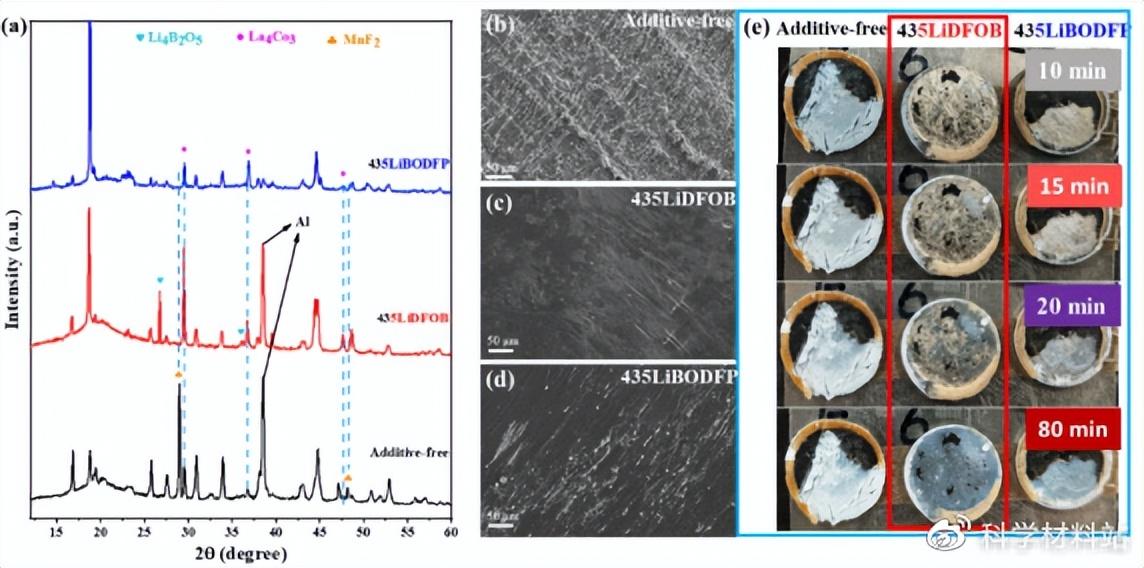
Fig. 3. (a) The XRD results of the positive electrode after 200 cycles at 1C; the surface morphology of the lithium negative electrodes assembled from (b) no additives, (c) 435LiDFOB and (d) 435LiBODFP composite electrolytes, respectively; (e) Photographs of the lithium anode exposed to air for different times after cycling.
Point 3: Functional additives can improve the electrolyte/negative electrode interface
The XPS test found that the functional additives participated in the formation of the SEI film on the surface of the lithium anode, thereby changing the composition and structure of the SEI film. Specifically, the SEI film formed by complete reduction of LiDFOB has a structure of organic lithium-containing layer/LiF-rich layer/Li2O-rich layer from the outside to the inside, and is uniform, thin and dense. In contrast, the SEI film induced by LiBODFP is defective because it is difficult to restore.
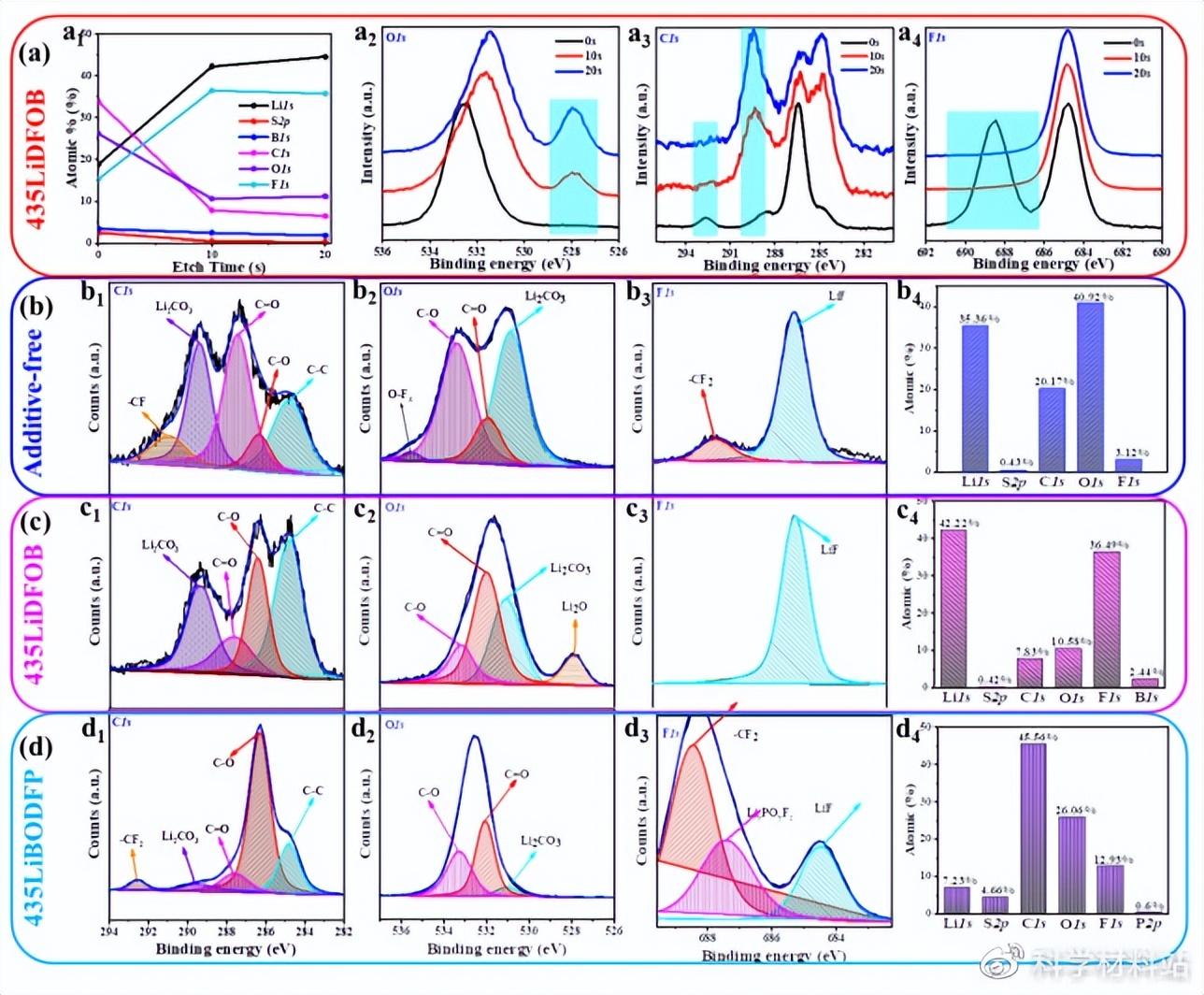
Fig. 4. (a) XPS scan results of cycled lithium anodes obtained from polycrystalline NCM622/435LiDFOB/Li cells at different etching times; composite solid electrolytes from (b) no additives, (c) 435LiDFOB and (d) 435LiBODFP XPS scanning results of the cycled lithium anode of the membrane-assembled battery when the etching time is 10 s.
Point 4: Functional additives can improve the cathode/electrolyte interface
Similarly, functional additives are also involved in the formation of the CEI film on the surface of the positive electrode. The easier oxidation of LiDFOB may lead to uneven distribution of decomposition products (such as LixBOyFz and LiF), and the CEI film induced by it is unstable. After long-term cycling, LixBOyFz can be completely oxidized to Li4B2O5 (confirmed by XRD results). therefore. The CEI film induced by LiDFOB cannot effectively suppress the side reactions at the cathode interface. In contrast, the CEI film induced by LiBODFP is stable, thin and uniform, and thus can effectively suppress the continuous occurrence of side reactions at the cathode interface.
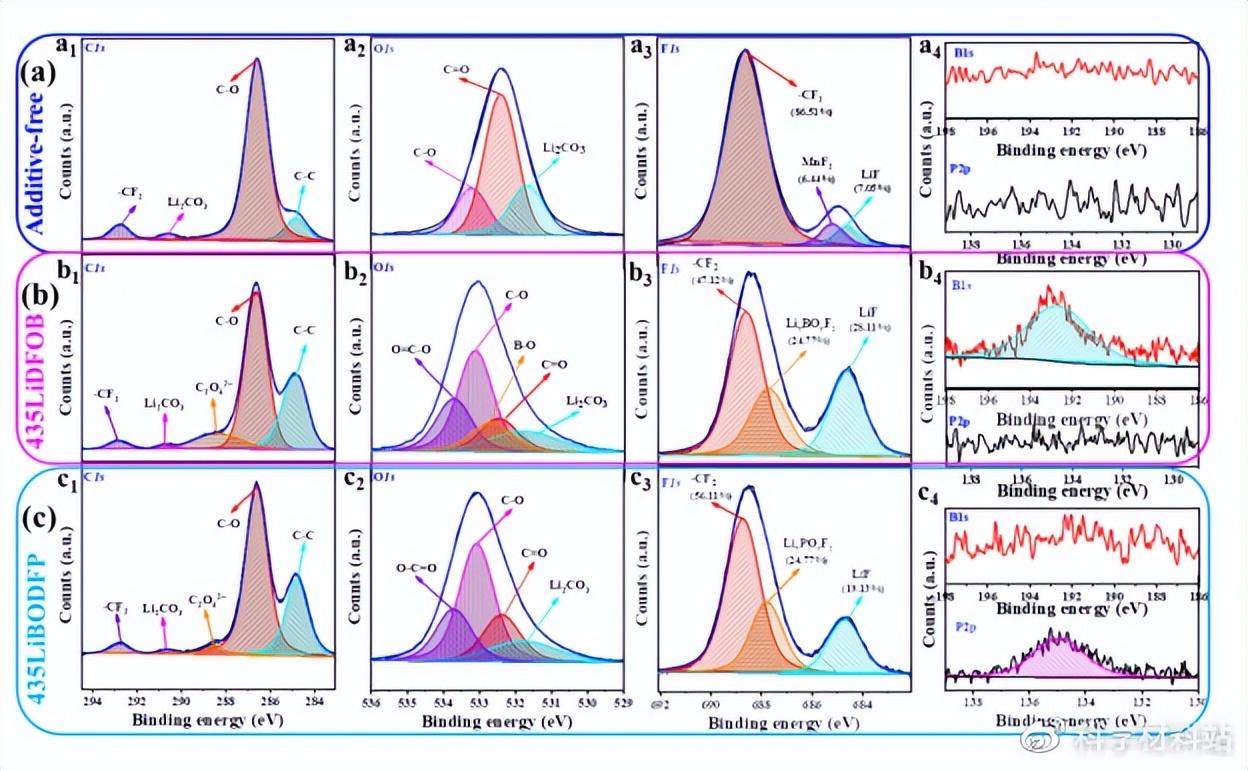
Fig. 5. XPS scan results of the positive electrode disassembled after cycling for batteries assembled from (a) additive-free, (b) 435LiDFOB and (c) 435LiBODFP composite solid-state electrolyte membranes.
Point 5: The combined use of bifunctional additives greatly improves the comprehensive electrochemical performance of all-solid-state batteries
Inspired by the above test results, a bilayer composite electrolyte was prepared to give full play to the role of LiBODFP and LiDFOB in stabilizing the positive and negative interfaces in all-solid-state batteries. The cycle performance of the battery assembled using the double-layer composite electrolyte membrane and the single-crystal NCM622 cathode material was significantly improved (Fig. 6c). Based on this, it can be concluded that the ultra-stable cycle of all-solid-state lithium metal batteries can be achieved by adding different functional additives on the positive and negative sides. This strategy will provide new ideas for the design and preparation of all-solid-state lithium metal batteries in the future.
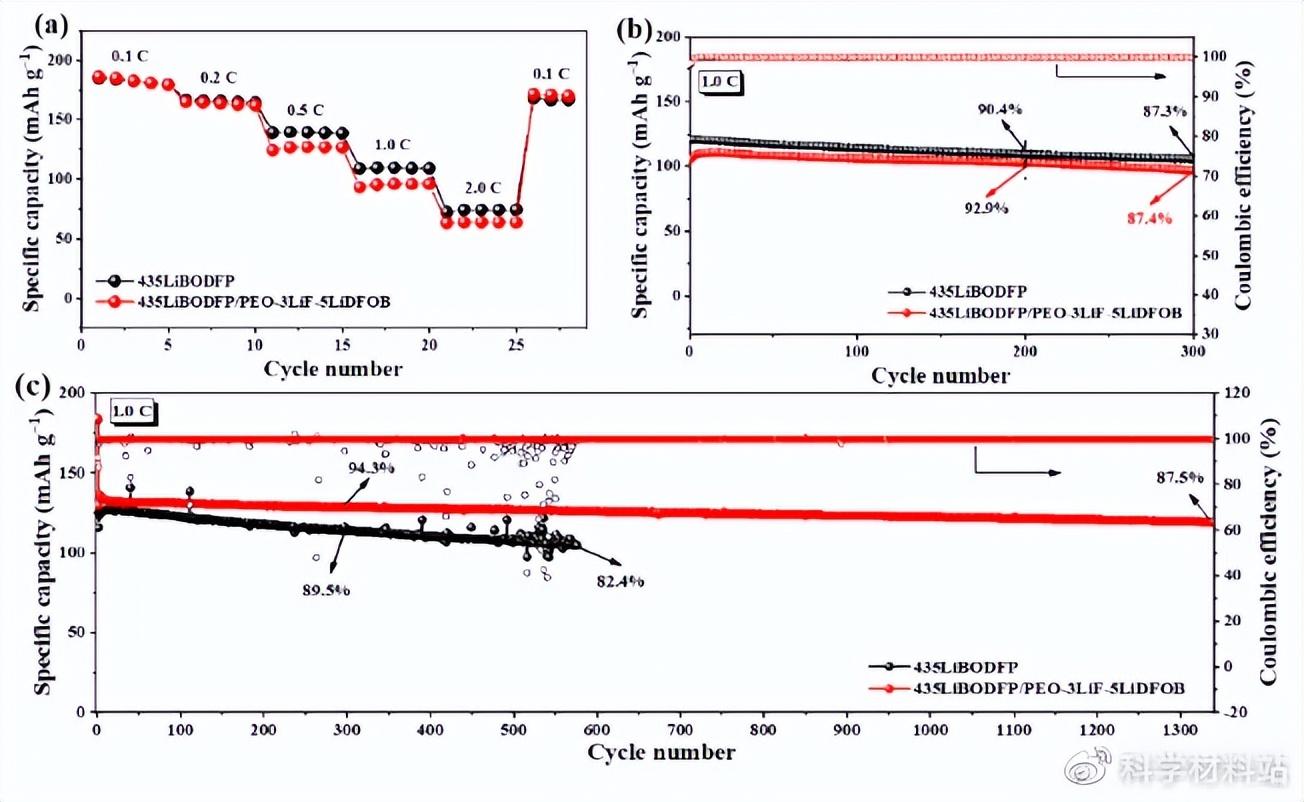
Fig. 6. (a) Rate performance of polycrystalline NCM622-based batteries assembled from single-layer and bilayer composite solid-state electrolyte membranes, (b) long-term cycling at 1.0 C of polycrystalline NCM622-based batteries assembled from different composite electrolyte membranes performance, and (c) the cycling performance at 1.0 C of single-crystal NCM622-based batteries assembled from single-layer and double-layer composite solid-state electrolyte membranes.
【Article link】
Optimized Functional Additive Enabled Stable Cathode and Anode Interfaces for High-voltage All-Solid-State Lithium Batteries with Significantly Improved Cycling Performance
https://pubs.rsc.org/en/content/articlelanding/2022/ta/d2ta03982f